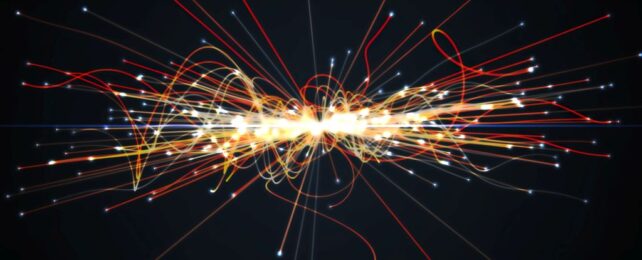
A rare type of particle has emerged from proton collisions in the Large Hadron Collider.
Between 2016 and 2018, physicists recorded more than 100 rare, unstable hypernuclei – atomic cores that contain an unusual flavor of quark in one of their nuclear particles.
It's a discovery that could help us understand the source of mysterious antihelium tentatively detected out in the great wide beyond of the cosmos.
The Large Hadron Collider is what it sounds like: a giant machine for smashing particles together in high-speed collisions, so that physicists can pore over the remains and look for things like unstable, short-lived particles that we can't detect any other way.
Nuclei and antinuclei – the hearts of atoms and their antiparticles – are fairly common, made up of baryons called protons and neutrons.
Baryons are in turn made up of a frothing mess of quarks and antiquarks, which are dominated on average by just three flavors; two up quarks and one down quark for protons, and one up quark and two down quarks for neutrons.
Far less common are hypernuclei, which contain hyperons in addition to protons and neutrons. In these baryons, strange quarks make an appearance.
Hypertriton is one of these hypernuclei; it consists of protons, neutrons, and Lambda hyperons, which contain one strange quark.
Hypernuclei such as hypertriton are of intense interest, not just for their own sake, but in an astrophysical context too.
Scientists think hyperons might form inside neutron stars, the collapsed cores of once-massive stars that have gone supernova. These cores are so dense that the physics therein is difficult to probe and understand.
But they also decay really quickly, so if we want to find hypertritons and their antiparticles, a particle collider is probably the only plausible place to look.
To find them, the Large Hadron Collider beauty (LHCb) collaboration used a new technique on data collected during one of the collider's previous runs. They didn't detect hypertriton or antihypertriton directly; rather, they found the products of its decay.
As the unstable particles fall apart, they transform into a cascade of lower-mass particles.
Here's what happens. Protons collide in the Large Hadron Collider, resulting in a release of energy that has a chance of producing a soup of particles.
In this rare case, a hypertriton or antihypertriton emerges, which flies for about 40 centimeters (16 inches) in about 240 picoseconds before decaying into an antiproton and a positively charged quark-antiquark pairing called a pion.
The pion flies out of the nucleus, but the antiproton remains trapped inside, transforming the antihypertriton into antihelium.
The process for the hypertriton goes the same way, except the hyperon decays into a proton and a negatively charged pion, and the nucleus is transformed into a plain old helium nucleus.
These pions and helium/antihelium nuclei are what the researchers detected in the Large Hadron Collider data, using a new technique for identifying helium, something the collider was not originally designed for. And by measuring the masses of the nuclei, the team was able to trace their formation to the decay of hypertritons and antihypertritons: around 61 of the former, and 46 of the latter.
The astrophysical implications are exciting. By gauging how antihelium is created and annihilated out in space, physicists can better constrain how much of it could feasibly be reaching Earth. This could validate or disprove that possible antihelium detection that was made back in 2018.
The discovery offers a new means for probing the properties of hypertritons. But the research has broader implications, too. The helium identification technique, the researchers say, also gives physicists a new tool for studying how the quarks in baryons are held together.
The findings have been presented at the European Physical Society Conference on High Energy Physics, and will be published in an upcoming paper.
0 Comments